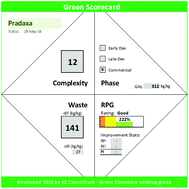
Green and sustainable drug manufacturing go hand in hand with forward-looking visions seeking to balance the long-term sustainability of business, society, and the environment. However, a lack of harmonization among available metrics has inhibited opportunities for green chemistry in industry. Moreover, inconsistent starting points for analysis and neglected complexities for diverse manufacturing processes have made developing objective goals a challenge. Herein we put forward a practical strategy to overcome these barriers using data from in-depth analysis of 46 drug manufacturing processes from nine large pharmaceutical firms, and propose the Green Aspiration Level as metric of choice to enable the critically needed consistency in smart green manufacturing goals. In addition, we quantify the importance of green chemistry in the often overlooked, yet enormously impactful, outsourced portion of the supply chain, and introduce the Green Scorecard as a value added sustainability communication tool.
http://pubs.rsc.org/en/Content/ArticleLanding/2017/GC/C6GC02901A?utm_medium=email&utm_campaign=pub-GC-vol-19-issue-1&utm_source=toc-alert#!divAbstract
The Green Aspiration Level (GAL) has been constructed on four pillars to ensure consistent application, namely (1) clearly defined synthesis starting points,1 (2) unambiguous complete E factor (cEF)2,3 or Process Mass Intensity (PMI) waste metrics, (3) historical averages of industrial drug manufacturing waste, and (4) complexity of the drug’s ideal manufacturing process (Supplementary Figure 6). cEF or PMI can be used interchangeably in GAL-based analysis enabling organizations using either to calculate their green performance scores. cEF and PMI differ by just one unit (Supplementary Equation 6) and share the same commercial waste goal for an average manufacturing step4 – the transformation-GAL or tGAL – that results in negligible numerical differences from the inclusion of one or the other. The pharmaceutical industry has generally adopted PMI. However, our publication utilizes cEF values due to literature prevalence and potentially broader appeal of E factors.5 It is important to note that all reaction and workup materials are included in the analysis, but excluded are reactor cleaning6 and solvent recycling.7 Standardized process starting points are a critical component of the GAL methodology. A starting material for some may be an intermediate for others. Until recently, the scientific community lacked an unambiguous definition of process starting points in the assessment of process greenness. This has been a bothersome source of inconsistency. Failure to define an appropriate starting material can lead to exclusion of significant amounts of intrinsic raw material waste created during earlier stages of manufacture. We therefore utilize these updated definitions of process analysis starting points to ensuring higher quality of data:8
1) The material is commercially available from a major reputable chemical laboratory catalog company, and its price is listed in the (online) catalog. Materials requiring bulk or custom quotes do not qualify as process starting material. AND 2) The laboratory catalog cost of the material at its largest offered quantity does not exceed US $100/mol. Therefore, published literature must be researched if the material does not qualify as process starting material in order to determine its correct intrinsic cEF. However, we realized that determination of literature cEF values is tedious and involves making assumptions since literature procedures are often incomplete compared to internal or external manufacturing batch records. Thus, standardizing Literature cEF quickly became a desirable goal. In order to facilitate literature analysis we introduced Supplementary Equation 7 that just requires determination of literature step count from ≤$100/mol starting materials without having to retrieve literature waste information.9 The literature step multiplier of 37 kg/kg represents the average literature step cEF across the analyzed projects (Supplementary Table 1), so it equals their average literature cEF (76 kg/kg) divided by average literature step count (2.1). The process cEF and Relative Process Greenness (RPG) derived from the simplified calculated cEF literature values are shown next to their progenitors in Supplementary Table 3. We observe that average calculated and manually determined cEF and RPG values are comparable and within 10% of their means across the three development phases. Thus, we consider the simplified method sound and an importtant element to achieving consistency in green process analysis.
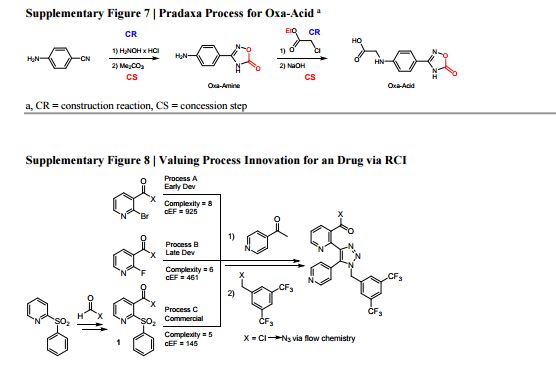
A deeper shade of green: inspiring sustainable drug manufacturing
Frank Roschangar,*a Juan Colberg,b Peter J. Dunn,c Fabrice Gallou,d John D. Hayler,e Stefan G. Koenig,f Michael E. Kopach,g David K. Leahy,h Ingrid Mergelsberg,i John L. Tucker,j Roger A. Sheldonkl and Chris H. Senanayakea
*Corresponding authors
bPharmaceutical Sciences – Worldwide Research & Development, Pfizer, Groton, USA
dChemical & Analytical Development, Novartis Pharma, 4002 Basel, Switzerland
eAPI Chemistry, GlaxoSmithKline Medicines Research Centre, Stevenage, UK
fSmall Molecule Process Chemistry, Genentech, a Member of the Roche Group, South San Francisco, USA
gSmall Molecule Design and Development, Eli Lilly and Company, Indianapolis, USA
hChemical and Synthetic Development, Bristol-Myers Squibb, New Brunswick, USA
iProcess Chemistry, Merck, Rahway, New Jersey 07065, USA
jProcess Development, Amgen, Thousand Oaks, USA
kMolecular Sciences Institute, School of Chemistry, University of the Witwatersrand, Johannesburg, South Africa
lDelft University of Technology, 2628 BL Delft, Netherlands
Green Chem., 2017,19, 281-285
DOI: 10.1039/C6GC02901A

Frank Roschangar, PhD MBA
Pharmaceutical process research director, passionate about accelerating drug development and driving green chemistry.
Ingelheim am Rhein, Germany
Research experience
-
Feb 2002–Sep 2015
Director
Boehringer Ingelheim
Germany · Nieder-Ingelheim
-
Aug 1996–Feb 1998
Postdoc
The Scripps Research Institute · Skaggs Institute for Chemical Biology · Prof. K.C. Nicolaou
United States · La Jolla
-
Aug 1992–Aug 1996
PhD Candidate
Rice University · Department of Chemistry
United States · Houston
Supplementary References
1. The $100 per mol laboratory catalog pricing requirement described in Supplementary Discussion 1 does not apply to reagents, catalysts, ligands, and solvents, since they are produced for widespread application and are not specific to the process being evaluated.
2. Since the original E factor has been applied inconsistently, the cEF metric was introduced for the purpose of GAL analysis. cEF accounts for all process reaction and process workup materials, including raw materials, intermediates, reagents, process aids, solvents, and water.
3. All E factors reported herein represent the cEF or sEF contributions of the overall manufacturing process or the sub-process (e.g. external cEF, literature cEF) to produce 1 kg of drug substance.
4. We define a step as a chemical operation involving one or more chemical transformations that form and/or break covalent or ionic bonds and lead to a stable and isolable intermediate, but not necessarily include its isolation. Examples: • Simultaneous removal of two or more protection groups involves multiple transformations, yet it is carried out in one chemical operation counted as one step • Sequential transformations via a stable and isolable intermediate that are carried out in two operations but without intermediate workup counted as two steps • Formation of covalent bonds or salts that occur during workup not counted as an extra step • Separate operation of salt formation from an isolated intermediate counted as one step • Isolation of a product, following work-up, as a solution that can be stored counted as one step.
5. A SciFinder search for the terms ‘Process Mass Intensity’, and ‘E factor’ and ‘Environmental impact factor’ on Nov. 14, 2016 revealed that the PMI concept was present in 12, 8, 9, and 12 publications for the years 2013-2016, respectively, while the E factor concept was mentioned 39, 45, 57, and 46 times (76-86%), respectively.
6. The GAL considers only direct process materials, i.e. materials used in the chemical steps and their workups. It does not include solvents and aqueous detergents required for reactor and equipment cleaning between batches or steps, nor the frequency and duration of the equipment and facility specific cleaning operations. These parameters are considered for comprehensive environmental impact in Life Cycle Assessment (LCA) analysis.
7. In US pharmaceutical manufacturing, recycling accounts for 25% of waste handling, while energy recovery burning and treatment constitute 38% and 35%, based on 2012 data from ‘The Right-To-Know Network’ (RTKNET.ORG), Toxic Releases (TRI) Database: http://rtknet.org/db/tri.
8. The $100 per mol commodity pricing criterion was established in ref. 15 of the main article based on the author’s professional experience. The authors of this manuscript consider this figure appropriate and helpful for providing a consistent analysis.
9. If a detailed procedure is available for a particular literature step, its calculated waste can be used in place of the 37 kg/kg default value.
10. J. Li and M. D. Eastgate, Current Complexity: a Tool for Assessing the Complexity of Organic Molecules. Org. Biomol. Chem. 2015,13, 7164–7176.
11. D. P. Kjell, I. A. Watson, C. N. Wolfe and J. T. Spitler, Complexity-Based Metric for Process Mass Intensity in the Pharmaceutical Industry. Org. Process Res. Dev. 2013, 17, 169– 174.
12. R. P. Sheridan, et al., Modeling a Crowdsourcing Definition of Molecular Complexity. J. Chem. Inf. Model. 2014, 54, 1604–1616.
13. M. F. Faul, et al., Part 2: Designation and Justification of API Starting Materials: Current Practices across Member Companies of the IQ Consortium. Org. Process Res. Dev. 2014, 18, 594–600.
14. Besides offering simplicity, the GAL’s process complexity model was selected vs. the alternative structural complexity measures due to its inherent ideality-derived consideration for available synthetic methodology.
15. See main article ref. 16: it defines Construction Reactions (CR) as chemical transformations that form skeletal C-C or C-heteroatom bonds. Strategic Redox Reactions (SRR) are construction reactions that directly establish the correct functionality found in the final product, and include asymmetric reductions or oxidations. All other types of non-strategic reactions are considered as Concession Steps (CS), and include functional group interconversions, non-strategic redox reactions, and protecting group manipulations.
16. M. E. Kopach, et al., Process Development and Pilot-Plant Synthesis of (2-Chlorophenyl)[2-(phenylsulfonyl)pyridin-3- yl]methanone. Org. Process Res. Dev. 2010, 14, 1229–1238.
17. M. E. Kopach, M. M. Murray, T. M. Braden, M. E. Kobierski, O. L. Williams, Improved Synthesis of 1-(Azidomethyl)-3,5-bis- (trifluoromethyl)benzene: Development of Batch and Microflow Azide Processes. Org. Process Res. Dev. 2009, 13, 152–160. 18. RCI (Process B) = 1 − ( ) = 0.25. RCI (Process C) = 1 − ( ) = 0.38
//////////green chemistry, drugs