Temperature control plays an important role in industrial processes, pilot plants, and chemical and pharmaceutical laboratories. When controlling reactors, both exothermic and endothermic reactions must be offset with high speed and reliability. Therefore, different conditions and effects must be taken into account when specifying an optimum and highly dynamic temperature control system.
.gif)
Temperature Control of Reactors
Most temperature control systems are used with chemical reactors made of either steel or glass. The former is more rugged and long-lasting, while the latter enables chemists to observe processes inside the reactor.
However, in the case of glass reactors, extensive precautions have to be followed for safe usage. Reactors usually include an inner vessel to hold the samples, which need temperature control. This inner vessel is enclosed by a jacket containing heat-transfer liquid. This reactor jacket is linked to the temperature control system.
In order to control the reactor’s temperature, the temperature control system pumps the heat-transfer liquid through the reactor’s jacket. Rapid temperature change inside the reactor is balanced by instant cool-down or heat-up, and the liquid is either cooled or heated inside the temperature control system. Figure 1 shows a schematic of a simple temperature control system.
.jpg)
Figure 1. Functional view of reactor temperature control
Process Stability
Both materials and reactor design can affect the temperature control of highly dynamic reactor systems. However, the heat transferred by a glass-walled vessel will be different than that transferred by a steel-walled vessel. In addition, both wall thickness and surface area can also affect accuracy. Therefore, proper mixing of the initial materials inside the reactor is important to obtain good uniformity, which in turn will guarantee optimal heat exchange.
For each type of reactor, maximum pressure values have been provided as per the specifications established by reactor manufacturers and in the Pressure Equipment Directive 97/23/EG. Regardless of any temperature control application, these limit values may not be surpassed during operation under any situations. Prior to starting a temperature control application, the applicable limits must be programmed within the temperature control unit.
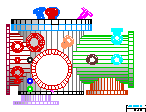
Another important criterion in reactors is the maximum permissible temperature difference, which is referred to as Delta-T limit. It defines the highest difference between the temperature of the contents of the reactor and the actual thermal fluid temperature.
When compared to steel reactors, glass reactors are more susceptible to thermal stress. For that matter, any temperature control system should enable users to program reactor-specific values for the Delta-T limit per time unit. Within the temperature control equipment itself, three components considerably affect the stability of the process and these include heat exchanger, pump, and control electronics.
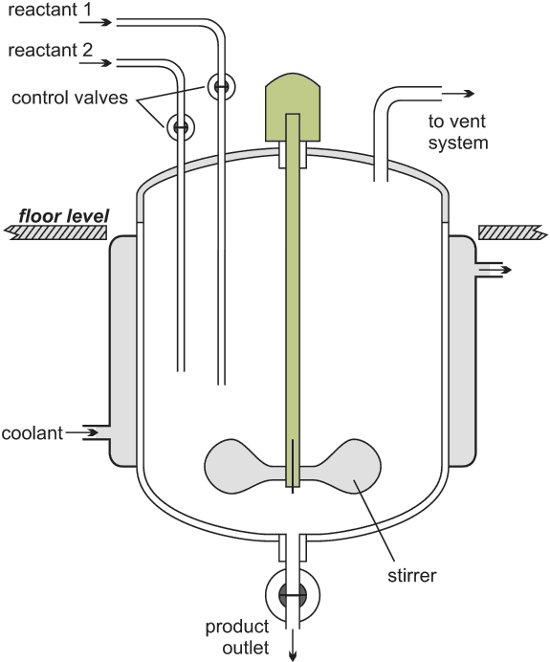
Heat Exchanger
It is important to ensure that a temperature control system has sufficient heating and cooling capacity, as this can significantly affect the speed to reach the preferred temperatures. In order to determine the preferred heating and cooling capacities, users must consider the essential differences in temperature, the volume of the samples, the preferred heat-up and cool-down times, and the specific heat capacity of the temperature control medium.
Highly dynamic temperature control solutions are commercially available in the market with water or air cooling. Air-cooled systems do not utilize water and may be deployed where there is sufficient air flow.
The heat thus removed from the reactor is eventually transferred to ambient air. Water-cooled systems need to be joined to a cooling water supply, but they operate more quietly and do not add surplus heat in small labs. These units could be completely enclosed by the application, if required.
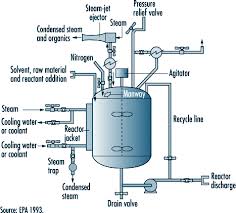
Pump
The integrated pump of the temperature control unit equipment must be sufficiently strong to obtain the preferred flow rates at stable pressure. To ensure that pressure limit values mentioned above are not exceeded, the pump should provide the preferred pressure quickly and with maximum control.
Operating conditions and pressure specifications of the reactor must always be taken into account, and regulation of pump capacity must be done by presetting a limit value. Sophisticated temperature control solutions include pumps that balance the variations of the viscosity of the heat transfer liquid to make sure that energy efficiency is maintained continuously.
This is because viscosity influences flow and hence the heat transfer. An additional advantage provided by magnetically coupled pumps is that they guarantee a hydraulically-sealed thermal circuit. Also, self-lubricated pumps are beneficial as they require only minimum maintenance.
The closed loop circuit prevents contact between the ambient air and the heat transfer liquid. This not only prevents permeation of oxidation and moisture, bit also prevents oil vapors from entering into the work environment.
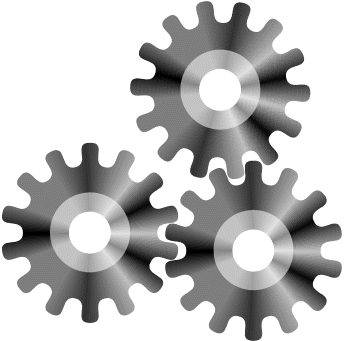
Additionally, an internal expansion vessel must permanently absorb temperature-induced volume variations inside the heat exchanger. Individual cooling of the expansion vessel will help in ensuring that the temperature control unit does not overheat and ultimately ensures operator safety.
A temperature control equipment should operate consistently even at high ambient temperatures. In majority of cases, the real work environment will diverge from the ideal temperature of 20°C. During hot summer months, temperature control solutions are exposed to adverse conditions. In laboratories, ambient temperatures are usually higher because of energy saving measures. These instances demonstrate the benefits of temperature control solutions that work consistently at temperatures as high as 35°C.

Control Electronics
Temperature control equipment includes advanced control electronics that monitor and control the process inside the reactor and also the internal processes of the system. When a control variable changes, the system is capable of readjusting the variable to the setpoint sans overshooting.
Accurate control electronics are needed to maintain the stability of a temperature control application. One option to assess control electronics is to look at the effort needed to set parameters. In a temperature control unit, users can enter a setpoint. Control electronics must be self-optimizing throughout the temperature control process to ensure optimum results.

Conclusion
To sum up, the process safety and stability during reactor temperature control relies on the effectiveness of heat transfer, the type of reactor, and the efficiency of the components within the temperature control unit. Therefore, different conditions and effects must be considered when specifying a highly dynamic temperature control system.

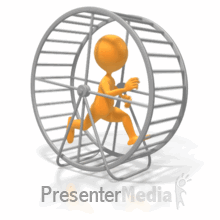